An Introduction
Compression molding is a common polymer manufacturing process for thermosets, thermoplastics, as well as elastomers and natural rubbers. It produces high-volume, dimensionally precise, high-strength, temperature resistant parts with good surface quality. Parts can be produced in an array of lengths, thicknesses, and complexities at a better per-part cost versus alternative manufacturing processes.
Parts manufactured in the compression molding process range from one ounce in weight to over 100 pounds and can mimic the strength and complex geometries of metal, doing so with anti-corrosive and electrically insulative properties. Products take many forms, from thin-walled containers to thick solid shapes including kitchenware, electrical housings, helmets, and airplane and automotive components.
Thermosets and thermoplastics come in a wide range of material options that differ in terms of price and performance. As such, compression molded Examples of resins and fibers used in the compression molding process:
Thermoset resins: SMC, BMC, TMC, epoxy, phenolic, melamine and urea.
Thermoplastic resins: polypropylene, nylon, UHMWPE, HDPE, and high temperature materials such as PEEK, PEKK and PAEK.
Fiber types: fiber glass, aramid, standard modulus carbon fiber, as well as other grades of carbon fiber.
How the materials perform directly correlates to how they are combined and the ratio of fiber to resin that is used, which results in different tensile strengths. For instance, the difference between thermosets like sheet molding compounds (SMC) and bulk molding compounds (BMC) is the way in which the fiber is chopped and mixed with the resin and the ratio of each materials used.
Material composition is of primary importance when establishing process parameters for compression molding. Material selection, in addition to part shape and thickness, will dictate the required preheating temperature, molding temperature, molding pressure, pressure holding time, cooling rate, exhaust pressure, exhaust times, and blank holder force.
Thermosets and thermoplastics differ in terms of their glass transition temperature (Tg), or the temperature at which the polymers reversibly transmute between glossy and rubbery states. The Tg for elastomers is below the ambient temperature, while thermosets and thermoplastics have a glass transition temperature that is above the ambient temperature.
Further to process parameters like temperature and pressure, material composition will impact the flow of the material. The impregnation effect of the reinforcing fibers will play a role in the mechanical performance and quality of the part being manufactured. Materials with more glass content will require greater pressure. The flatter and simpler the geometry, less time and pressure will be required to mold the component.
For example, running a 17 to 20 percent glass content versus a 40 to 50 percent glass content will require less pressure to encourage material flow. High glass content materials can be offset by incorporating a charge with greater coverage, which results in a process closer to stamping.
Engineers can determine the process parameters including the appropriate amount of material required to produce the desired part, the minimum amount of time and energy required to heat the material, the appropriate heating technique, and the required amount of force to optimize the process and output, though there are some guidelines that can be followed.
As a general rule of thumb to calculate the required tonnage:
W" x L" x PSI x 0.00046 = Tons
Width (in inches) x Length (in inches) x 2000 pounds per square inch x 0.00046 = Tonnage Required
Process controls should also be designed and integrated with incremental capabilities, to allow the process to step into different tonnages at different positions, especially where complex geometries are concerned. It is ideal to incorporate at least five different settings in the controls. The molder can determine these parameters.
The Compression Molding Process
Compression molding requires a mold, which will be machined from steel or aluminum and will be designed to satisfy dimensional and tonnage requirements. As explained in the introduction, a general rule of thumb to calculate the required tonnage is to use the following formula: Width (in inches) x Length (in inches) x 2000 Pounds per Square Inch x 0.00046 = Tonnage required.
As material properties are of great importance in the compression molding process and the establishment of process parameters, the process for thermosets and thermoplastics are similar but also differ in important ways.
The compression molding process for thermosets:
-
The mold, or metal tooling, comprised of a cavity and a core, is installed in the press, and may feature integral heating to control temperatures throughout the process. Temperature can also be controlled from the platens of the hydraulic press.
-
Once the tool is installed and heated, fiber reinforced resin material, or the charge, is placed in the tool. The size, weight and orientation of the polymer charge will have implications on the performance of the part when completed. In some cases, the charge is pre-formed or pre-heated.
-
Once the material is in the heated cavity of the mold, the press closes at a programmed speed and pressure. When the two mold halves come together, they force the material through the entirety of the mold cavity to produce a part. The tool will remain closed, under pressure, for a predetermined amount of time based on the process requirements of the material, causing an irreversible chemical crosslink to occur which results in a cured part.
-
Once the part is cured, the pressure is released, and the part is removed from the tool. There may be a small amount of resin flash that will need to be removed.
-
Thermosets require tighter temperature controls to prevent defects and warping. Rapid cycle times prevent the material from the risks associated with pre-cure if it stays in the heated mold for too long.
Process parameters – thermosets:
Clamp Tonnage 1000 – 2500 psi on the molded surface area of the part
Clamp Close Speed 500 – 1200 inches per minute
Pressing Speed 0 – 35 inches per minute
Temperature 290 to 400°F
The compression molding process for thermoplastics:
For thermoplastics, the process is relatively the same. The major difference is, in the case of thermoplastics, temperature controlled cooler molds are used to promote the hardening of the material. Once hardened, it can be removed from the mold. The process also varies depending on the material selected
Process parameters – thermoplastics:
Clamp close speed: Clamp close speed can go to as high as 1,900 inches per minute. Speeds are greater than those required for thermosets because the press has to come down on the material at a rate that will prevent pre-cooling or precure. Preheating and premeasuring reduces excess flash, but also helps to prevent precure.
Clamp Tonnage: Ensuring adequate clamp tonnage will improve output by reducing part defects and pre-mature machine breakdown, saving money in the process. If your process requires 200-tons of clamping force, a 400-ton machine is excessive. It is important to identify a capable machine for your materials and processes and a press manufacturer that has the engineering expertise to design a press that is built to perform.
Pressing speeds: Thermoplastics require a greater range of pressing speeds. The cycle can last anywhere from a few minutes to an hour, depending on the material selected, the size of the charge and the time required for cooling and curing. Fast pressing cycles, with pressing speeds upwards of 190 inches per minute, promote optimal production output and prevent any buildup of residual stresses in the parts from occurring which can lead to warpage, fatigue and cracking.
Temperature: Tools are heated to between 95 and 180°F when compression molding thermoplastics. Materials will come out of the press at temperatures between 450 and 550°F. Unlike thermoset parts, thermoplastic parts do not crosslink, so they can be re-melted and reused without changing the chemical composition of the material. They are processed in a molten state and the cooling process establishes the internal structure and shape.
Press Features
Compression molding presses can feature enhancements and process controls such as temperature compensated adjustable guiding, part handling, feed and die automation, core and ejector packages, platen locking devices, mold and platen heating packages, direct or accumulator hydraulic drives, intelligent platen, parallelism and vaccuum packages.
Intelligent levelling systems, as well as parallelism, are especially important for processes that include in-mold coating or situations with off-center loading. Servo-proportional controlled levelling cylinders, powered levelling spindles and associated electronic control packages can be built into presses to ensure platen parallelism and ultimately, maximized performance and output. Vaccum packages reduce void content if an issue with gases in the material arises, causing voids in the molded part.
Likewise, precise platen control is imperative to repeatable success in part production. Smooth pressing cycles and adjustable pressing speeds control the rate of material flow in the cavity and prevents any grain in the final product.
Thermosets in the Compression Molding Process
Thermoset composites are the most common type of material used in the compression molding process. Thermosets include Bakelite, polyester, polyurethane, urea-formaldehyde, as well as melamine, epoxy, and alkyd resins.
Thermoset materials are selected based on their properties, the application, and the associated costs. For example, vinyl ester resin utilized for its for corrosion resistant applications, while epoxy resin satisfies the design requirements of high-strength applications and polyester resin is usually selected for both cost and performance factors.
Utilizing thermosets in the compression molding enables manufacturers to achieve complex geometries and parts that mimic the properties of metal with several additional advantages. The strength-to-weight ratio it offers is ideal for applications like automotive parts, as they produce lighter, durable components that will not add significantly to the weight and performance of the vehicle.
While strength and structural integrity are certainly competitive advantages of thermosets, its downfalls include poor elasticity and elongation. Its low initial viscosity can result in flash and the need for secondary machining operations. It also tends to be more difficult to surface finish. Likewise, high levels of some fillers can lead to excessive tool wear.
When molded, the resulting structure of the material is highly crosslinked, comprised of heavily branched molecules, chemical bonds that promote high mechanical and physical properties. As a result, it cannot be recycled like its thermoplastic counterparts. Thermosets are selected where the strength and quality of the part is dependent on the degree of crosslinking that takes place.
Thermoset polymers undergo three distinct stages:
Stage one: During the first stage, also known as resole, the resin is still fusible and soluble.
Stage two: In the second stage, the thermosets are almost insoluble but are thermoplastic. They will only stay in this molten state for only a short period of time as the temperatures promoting flow of the material will cause cross-linking.
Stage three: The final stage of polymerization is where the cross-linking reaction takes place, under controlled impact of heat and pressure.
Thermoset is typically selected because of its material properties. It is not prone to swelling and it is insoluble in the presence of most organic solvents. It also offers a high resistance to creep, low thermal conductivity, microwave transparency and exceptional dielectric strength, as well as the option of molded-in tolerances, color, and surface finish varieties.
As the compounds used in the material are reactive systems, they can impact the shelf life of the material and could lead to variation in the batch processes in terms of consistency on a lot-to-lot basis. Thermosets will require temperature-controlled storage, an added consideration in the material selection process.
Other process parameters that will have implications on the mechanical properties and performance of thermosets in the compression molding process is compression temperature, the pressure during compression, the pressure holding time, the cooling rate and mold opening temperature, as well as preheating temperatures, exhaust temperatures and pressures.
When the process and material selection in the compression molding of thermosets are ideal, it promotes functional designability, simple fabrication, fast processing, and high cost performance at a good price point.
Commonly Used Thermosets
Epoxy, phenolic and unsaturated polyester resins, unsaturated polyester, vinyl ester, polyurethane and anaerobic adhesives are all examples of commonly used thermosets in the compression molding process.
Below, the two photos highlight the difference between preformed epoxy material and phenolic material:
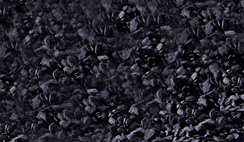
Sheet molding compound (SMC)
Sheet molding compound (SMC) is a fiber reinforced thermoset material (a combination of polymer resin, inert fillers, fibers, catalysts, pigments, stabilizers, release agents and thickeners) often used for larger parts that require greater mechanical strength. Glass reinforcement is between 10 and 60 percent and fiber length is slightly longer than those of bulk molding compound (BMC), between ½-inch and 1-inch.
The manufacturing process of SMCs is a continuous in-line process where the material is sheathed both top and bottom with a polyethylene or nylon plastic film to prevent auto-adhesion. The paste is spread uniformly onto the bottom film, the chopped fibers are added on the paste in a random fashion and the top film is introduced to the process, rolled into a pre-determined thickness and left to mature for 48 hours.
SMC satisfies applications that produce appearance critical parts that have a Class A finish, but it is also ideal for painting and structural applications that require strength and stiffness, but not an appearance critical aesthetic.
Bulk molding compound (BMC)
Bulk molding compound (BMC) is a thermoset plastic resin that is similar to SMC but the way in which the fibers and the resin are combined differs. It is still a blend of inert fillers, fiber reinforcement, catalysts, stabilizers, and pigments, but they form a viscous putty. The material is highly reinforced using short glass fibers, with a glass reinforcement measure of 10 to 30 percent, with lengths between 1/32-inch and ½-inch.
BMCs will achieve close dimensional control, flame and track resistance, electrical insulation, corrosion and stain resistance, in addition to heightened mechanical properties, minimized shrink capacity, and color stability which make it ideal for applications requiring precision in detail, dimension and performance. It can also tolerate powder coating and water-based paint.
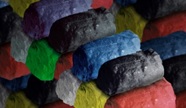
Thermosets, in general, offer excellent flame, smoke and toxicity characteristics. A great example is cyanate ester which boasts extremely low out gassing attributes. They also promise low density, corrosion resistance and dielectric characteristics. They are cost-effective and offer design flexibility that many projects yearn for.
Composite materials
Composites, such as laminated plastics, are also thermosets that are utilized in the compression molding process. Laminated plastics are a special form of polymer-matrix composite that is comprised of layers of fiber reinforced materials that are impregnated with thermosetting resins (typically phenolics), bonded together using heat and pressure.
Once the material is impregnated and dried, it is cut into sheets, stacked together, and pressed using high pressure and specified temperatures (270° to 350°F). This results in a laminated composite that can range from hard materials to softer plasticized grades, depending on the application.
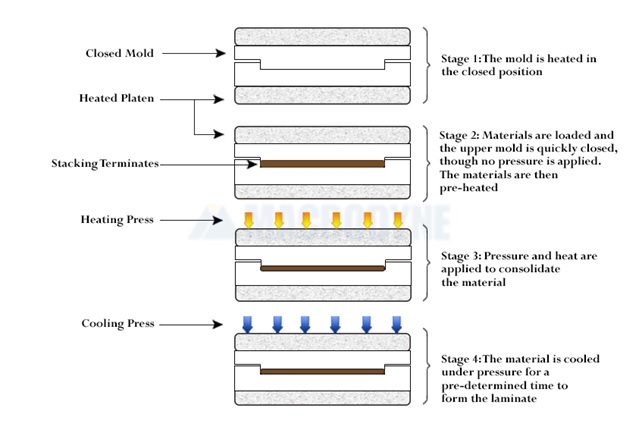
Laminated thermosets offer high mechanical strength, dimensional stability, rigidity, a high strength-to-weight ratio, as well as good electrical, moisture and temperature resistance. During the production process of these multilayer materials, it is important that fibers are thoroughly wetted with resin, with the excess resin removed.
Laminate needs to be consolidated to thickness and all trapped air, moisture and solvent vented to avoid porosity in the material. Curing time is imperative to ensure the material is neither over-, nor under-cured.
Applications for these laminated composite materials include switchboard panels and terminal boards, starter/generator/television insulation, gaskets, washers, gears, or any other product where high feeding and cutting speeds are present.
Cured laminates, also referred to as high-pressure laminates, are produced in over 70 standard grades in compliance with National Electrical Manufacturers Association (NEMA) specifications. The same base materials can be found in molded-laminates and molded-macerated parts where mold costs are justified by production quantities and where machining from flat laminates is uneconomical.
Thermoplastics in the Compression Molding Process
Just like its thermoset counterpart, thermoplastics offer a great alternative to metal parts where corrosion is a concern. It can stand up to harsh, even toxic environments, and offer a much greater finish for the associated costs when compared to alternative materials.
There are two categories of thermoplastic polymers: amorphous and semi-crystalline. Amorphous thermoplastics form no crystalline structure. Above the glass transition temperature (Tg) the polymer modules are solids and enough energy exists to promote movement of the molecules in relation to each other, enabling molding to take place at these temperatures.
Poly-ether-imide (PEI) is an example of an amorphous thermoplastic polymer. Its Tg is 423°F and its process temperature ranges between 575° and 625°F. Nylons can be amorphous or semi-crystalline. Unlike semi-crystalline polymers, amorphous thermoplastic polymers can be molded at temperatures closer to their Tg.
Semi-crystalline polymers have areas where the polymer packs closely together and forms a crystal lattice, as well as areas where it is amorphous. The degree of crystallinity in a specific part will depend on the polymer type and the rate of cooling. Poly-ether-ether-ketone (PEEK), poly-ether-ketone-ketone (PEKK) and Poly-phenylene-sulfide (PPS) are all semi-crystalline thermoplastic polymers.
PEEK: Tg - 290°F, process temperature range - 715°F to 740°F.
PEKK: Tg - 312°F, process temperature - 645°F to 690°F.
PPS: Tg - 192°F, process temperature – 550°F to 620°F.
Further to their categorical classification, thermoplastics exist in three material forms. The first are the fabric prepregs that employ a common carbon or glass fiber woven material with a thermoplastic resin incorporated into the fabric.
They are also referred to as semi-pregs because the resin is mostly on the surface until high temperatures are introduced to initiate the impregnation process. These conformable materials are used primarily on large continuous structures.
The second material form refers to reinforced thermoplastic laminates (RTL), multi-ply oriented laminates that vary in terms of the materials used and the number of layers they form, as well as width and length and are classified as such, on the basis of grade, class, resin and orientation.
RTLs have undergone the high-pressure, high-temperature thermoforming process to achieve optimal fiber bundle impregnation of the thermoplastic resin. Given the ability for RTLs to be quickly heated and processed, it is utilized in short cycle thermoforming processes.
The third material form in which thermoplastics exist is a thermoplastic unitape that ranges in width, including chopped molding compound grade, or one-eighth-inch slit tape. The advantage of this form is efficiency when using automated tape laying and fiber placement equipment and the wide variety of automation solutions that exist.
When compared to thermosets, thermoplastics come with a higher initial raw material cost over thermosets and increased tooling costs due to the higher processing temperatures required. Unlike thermosets, which require cold storage and cold transportation, there is no chemical reaction in thermoplastics so they can be stored at room temperature with no degradation of its properties.
Other advantages of thermoplastics include high strength, shrink-resistance, and flexibility. Thermoplastics are applicable for use in low-stress applications or high-stress mechanical parts. The weight to performance ratio of thermoplastics, as well as its versatility and recyclability make it ideal for high volume, precision applications. Unlike thermosets, its curing process is reversible, as no chemical bonding takes place. When recycled and remolded, its physical properties are not compromised.
The primary disadvantage of using thermoplastics instead of materials such as metal, is their relatively low melting point. Certain types of low-quality thermoplastics can melt when they are exposed to the sun for extended periods. Some thermoplastics can also have poor resistance to organic solvents and hydrocarbons.
Another disadvantage of thermoplastics is that they are susceptible to creep, which occurs when the material stretches and weakens under exposure to long-term stress loads. The susceptibility to creep is further exacerbated by the lower melting temperature of the material. Other types of thermoplastics, such as composites, can fracture instead of becoming deformed under high-stress conditions.
Commonly Used Thermoplastics
Commonly utilized thermoplastics include polyethylene (PE), polyvinyl chloride (PVC), and polystyrene (PS), as well as acrylics, fluoropolymers, polyesters, polyimides, and nylons. They are used in a number of applications from plastic containers to bottles, plastic bags, ropes, belts, components for aerospace applications, medical devices, and many more.
PEEK is a high performance, semi-crystalline, organic thermoplastic polymer that is used in a number of engineering applications. It is colorless and organic and part of the polyaryletherketone (PAEK) family. As an advanced biomaterial, PEEK exhibits excellent mechanical and thermal properties, resistance to creep at high temperatures, low flammability, and resistant properties.
PEEK’s mechanical and chemical resistance properties are retained in high temperatures and organic and aqueous environments, making it ideal for applications such as bearings, piston parts, pumps, compressor plate valves, electrical cable insulation, used in automotive, aerospace, chemical processing and many other applications. It is also used in the production of medical devices and implants.
Due to the fact that PEEK melts at a relatively high temperature, it results in parts that are thermostable and electrically and thermally insulated. Like other semi-crystalline materials, it is subject to dimensional changes and shrinkage when it crystalizes, though it can be easily accounted for in the process.
Another material in the PAEK family is PEKK. Also considered a high-performance semi-crystalline thermoplastic polymer, it too provides strength, heat and chemical resistance and low flammability, ideal for demanding applications. Though PEKK is similar to PEEK, the former replaces one of the flexible ether linkages in the material composition making it a more rigid ketone offering.
PEI is an amorphous thermoplastic with high mechanical, thermal and electrical properties, strength, and rigidity. When reinforced with glass fiber, it provides greater tensile strength, rigidity, and improved dimensional stability.
As a plastic, it possesses similar characteristics to polyarylsulphones (PSU, PPSU), though it is similar in benefits. It offers resistance to creep over a wide temperature range, hydrolysis, and dimensional stability at a high permanent operating temperature, as well as electrical insulation, resistance to radiation, chemicals like chlorine and other caustic cleaning agents.
High-density polyethylene (HDPE) is another commonly used thermoplastic. It is derived from the natural gas ethane, which, when heated to 1500°F, its molecules break apart. One of the separated molecules is the gas ethylene which becomes a resin in the polymerization process and produce polyethylene. Polyethylene is used to make a number of different types of plastics including low-density polyethylene (LDPE) and polyethylene terephthalate (PET or PETE).
HDPE’s properties make it resistant to many solvents, impact and weather resistant, it has a high density-to-strength ratio, meaning it is lightweight and strong, and it is ideal for recycling and reuse which is why it is frequently the material of choice for bottles or bags, as well as toys, pipes, lumber and fireworks.
Direct long fiber thermoplastic (DLFT) are essentially long fiber thermoplastics (LFT) in direct compounded form. It is a type of composite material where thermoplastic polymers are used, mixed with fiber reinforcements and other additives, molded, and cured under pressure to produce a strong material that offers geometric flexibility and faster cycle times.
In the case of DLFT, size does matter. It is ideal for large automotive parts, as the smallest parts should weigh at least 2 pounds, though 4 pounds or greater is preferred. It can be used where decreased weight and increased performance are desired material properties. It can be used to produce decorative surfaces with textures requiring minimal finishing.
While DFLT can be recycled and reused, without compromising its performance. Disadvantages include higher tooling costs limited heat resistance, limited true class A capability and weaker dimensional stability when compared to thermosets.
As indicated, there are countless resin and fiber combinations that result in a diversity of materials and material properties that can be utilized in the compression molding process, which is why it is important to leverage the knowledge and support of experts who can lead you through the process and material decisions to ensure your application and output performs optimally and produces desired results.
Advantages and Disadvantages of Compression Molding
Compression molding produces composite parts of varying complexities that are comparable to those manufactured from metal. They offer the same strength and mechanical properties but outperform metals in their weight-to-performance ratio, anti-corrosive and electrically insulative properties, and require less post-fabrication machining to meet geometric specifications.
One of the greatest advantages of the compression molding process is the ease at which ribs, and other inserts can be incorporated at the time of forming which reduces or completely eliminates the need for secondary processes. With one process, it can replace several assembled parts with one complex compression molded part.
The ability to incorporate secondary processes reduces the need for labor and parts inventory and minimizes material scrap and inspection times. Another benefit is the low tooling cost and the need for less costly capital equipment, as well as the fact that it is adaptable to automation. All of these things result in cost savings without compromising performance, quality, and output.
Shortfalls of compression molding are that the process is not ideal for very intricate parts or parts containing undercuts, side draws, or small holes, as it runs the risk of producing small distortions or breaks as a result of the process flow and pressure.
From a process perspective, compression molding rivals injection molding in most ways, except in terms of cycle times which are longer on account of heating and cooling processes, which are paramount to part performance and quality when using thermosets and thermoplastics in the compression molding process.
To learn more, visit our website at www.macrodynepress.com/hydraulic-presses/compression-molding-presses